SPECIAL FEATURE Toe buckling deformation in Central Otago Schists
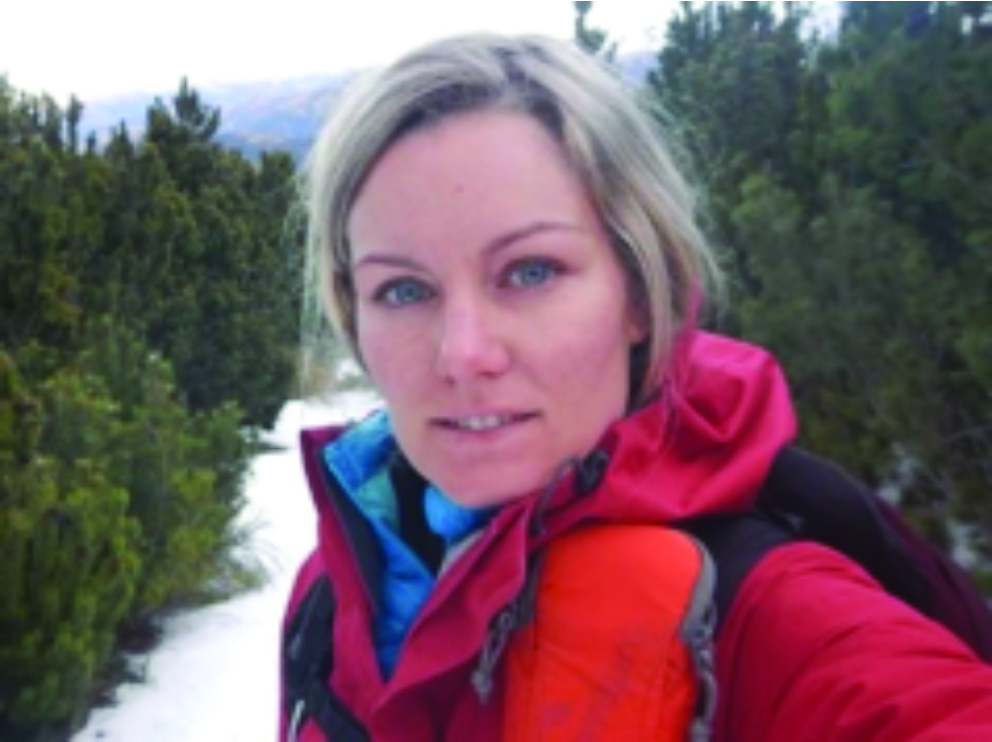
Figure 1: a) and b) Incision of the valley induces a progressive rotation of principal stress axes (σ1, σ3); c) once accumulated stress concentrated at the base of the slope exceeds the strength of the anisotropic rock, toe buckling deformation develops in the form of oversteepened foliations.
1. Introduction
Toe bucking deformation is not a widely familiar mechanism within the field of rock mechanics. Case studies, however, indicate that this type of deformation has been identified globally in a wide range of environments as a result of anthropogenic infrastructure such as road cuttings and open pit mines (Hu and Cruden 1993; Stead and Eberhardt 1997) and natural large scale landslides associated with deep seated gravitational slope deformations (DSGSD) (Agliardi et al. 2001). In some instances, toe buckling has triggered catastrophic landslides, such as during the 1999 Chi Chi earthquake in Taiwan (Wang et al. 2003). It is therefore important for hazard management and civil engineering design applications to identify and understand the major parameters governing this type of deformation mechanism.
Research in the form of a PhD project is currently in progress, focusing on toe buckling deformation in a New Zealand context. The study is based on three large scale landslides in Cromwell Gorge, Central Otago, which exhibit toe buckling deformation beneath their basal failure zone. Kinematic instability of these slopes are considered to be related to toe buckling deformation. It is anticipated that mass movement was triggered in response to breakout at the toe buckled hinge zone. This theory is being verified through numerical back analysis of the evolution of Cromwell Gorge.
2. What is toe buckling deformation?
Toe buckling deformation is associated with the development of slopes either through anthropogenic excavation or via natural processes such as uplift and erosion, where removal of the overburden induces the localised rotation of principal stress axes, as demonstrated in Figure 1. Differential stresses concentrated at the base of the slope can lead to the outward expression of strain in the form of toe buckling deformation, where stresses exceed the strength of an anisotropic rock mass. Variations of toe buckling deformation comprise curvilinear flexural buckling and three hinge buckling (see Cavers, 1981).
3. Location and background
Toe buckling deformation has been identified beneath the slide bases of several large scale landslides in both the Kawarau and the Cromwell Gorges located in Central Otago, New Zealand (Beetham et al. 1991b). This research focuses on the landslides within the Cromwell Gorge due to the detailed geotechnical database available from the Clyde Hydropower Project. Between 1975 and 1992, the Cromwell Gorge was subjected to extensive geotechnical works whereby seventeen major landslides were identified and subsequently stabilised in the Lake Dunstan Catchment (Macfarlane 2009) (see Figure 2).
Figure 2: Locality map of Cromwell Gorge depicting approximate locations of major landslides within the Lake Dunstan catchment (modifed from Macfarlane 2009). The zone of observed toe buckling deformation is highlighted in red.
The results of these extensive geotechnical investigations indicated that in general, the Cromwell Gorge comprises Haast Schist (textural grade IV) bedrock, which is inclined at an average angle of 20° to 40° towards the southwest. Anomalous, oversteepened to overturned foliations were identified beneath the slide bases of three landslides, namely Nine Mile, No. 5 Creek and Dunlays Slides (Beetham et al. 1991b). This zone of deformation was identified along the Dunstan Flank of the gorge as highlighted in Figure 2. Bedrock in this area is deformed with schistosity exhibiting flexural buckling steepening upwards toward the slide base at angles from 50° to 90° to completely overturned (Figure 3).
Figure 3: Inferred geological cross section of Nine Mile (Downstream) Slide illustrating deformed schist directly underlying the basal failure zone comprising oversteepened to overturned foliations (modified from Beetham et al. 1991a).
4. Numerical Modelling
Toe buckling deformation identified along the Dunstan Flank is considered to be a result of time-dependent gravitational creep deformation in response to progressive incision of the Cromwell Gorge. Finite Element Method (FEM) numerical models were used to model the anisotropic nature of the schist rock mass by incorporating an elasto-plastic Mohr-Coulomb failure criterion with an explicit joint network. A sequential unloading method (Figure 4) was adopted to simulate valley evolution commencing from a relatively low relief surface, corresponding to the Otago Peneplain, progressively incising through uplift and erosion to present day topography (1400 m deep valley). A summary of the FEM input parameters and model considerations are provided in Table 1.
Table 1: Summary of input parameters and model considerations.
Figure 4: Sequential unloading method adopted in FEM model where staged excavations correspond to suggested exhumation rates for the Cromwell Gorge commencing from a low relief surface (Otago Peneplain) progressively incising to the present day topography (~1400 m deep valley).
5. Preliminary results
Preliminary results suggest that the major parameters which governed the development of toe buckling deformation in the Cromwell Gorge are the combination of topographically induced gravitational stresses, high far-field active horizontal tectonic stresses, foliation stiffness and orientation. Flexural “z-shaped’ folds (toe buckling) develop in a shear band along a linear zone of abrupt principal stress orientation change. This shear band, shown in Figure 5, develops at the intersection of topographically induced gravitational stresses localized proximal to the slope (red stress trajectories) and the far field horizontal tectonic stresses which dominate along the base of the slope (black stress trajectories).
The anisotropic nature of the schist plays an integral part in the development of toe buckling. Numerical simulations reveal that ‘z-shaped’ folds (toe buckling) do not develop where foliations are orientated sub horizontally to gently inclined (10-20°) or sub vertically inclined (80°). In addition, a certain magnitude of foliation competency (stiffness) is required to facilitate buckling. At low assigned shear and normal stiffness, the material merely yields in translation along preferential planes of weakness (foliation shears). Whereas, at higher stiffnesses, foliations facilitate plastic deformation by withstanding complete dislocation, and deform in a curvilinear manner (i.e. ‘z-shaped’ buckle folds).
Figure 5: Foliations deform via flexural buckling developed along bands of maximum shear strain due to localised rotations of principal stress axes between topographically induced gravitational (red stress trajectories) and far field tectonic principal stress (black stress trajectories) orientations. Note the height of the slope was scaled from 1400 m to 400 m for model simplification.
6. Conclusions
Back analysis of toe bucking deformation in the Cromwell Gorge using FEM numerical simulations of valley evolution indicates that oversteepened foliations at the base of the Dunstan Flank are a product of prolonged stresses acting on an anisotropic rock mass. Preliminary results reveal that dominant contributing parameters to this style of deformation are topographically induced gravitational stresses, high horizontal tectonic stresses and the anisotropic nature of schist (foliation orientation and stiffness).
Moving forward with this research, further geotechnical laboratory tests will be carried out on Rakaia Terrane Schists (a subdivision of Haast Schist) as preliminary research highlighted limited availability of published geotechnical data. Additional numerical simulations incorporating new laboratory data as well as creep constitutive models will be processed. Furthermore, structural mapping and the implications of potential imbricate nappe-like structures associated with the juxtaposition of the Caples Terrane over the Rakaia Terrane will be considered. Any comments or points of consideration for this research will be welcomed. Please contact Romy Ridl on romy.ridl@pg.canterbury.ac.nz
7. References
Agliardi, F., Crosta, G. & Zanchi, A., 2001. Structural constraints on deep-seated slope deformation kinematics. Engineering Geology, 59, pp.83–102.
Beetham, R. et al., 1991a. Clyde Power Project. Nine Mile Slide (Downstream) engineering geological assessment investigations and remedial works – Addendum B, New Zealand Geological Survey: Department of Scientific and Industrial Research: Geology & Geophysics.
Beetham, et al., 1991b. Landslide development in schist by toe buckling. In D. H. Bell, ed. Landslides. Rotterdam: Balkema, pp. 17–23.
Cavers, D.S., 1981. Simple methods to analyze buckling of rock slopes. Rock Mechanics, 14, pp.87–104.
Hu, X.-Q. & Cruden, D.M., 1993. Buckling deformation in the Highwood Pass, Alberta, Canada. Canadian Geotechnical Journal, 30, pp.276–286.
Macfarlane, D.F., 2009. Observations and predictions of the behaviour of large, slow-moving landslides in schist, Clyde Dam reservoir, New Zealand. Engineering Geology, 109, pp.5–15.
Stead, D. & Eberhardt, E., 1997. Developments in the analysis of footwall slopes in surface coal mining. Engineering Geology, 46, pp.41–61.
Wang, W.N., Chigira, M. & Furuya, T., 2003. Geological and geomorphological precursors of the Chiu-fen-erh-shan landslide triggered by the Chi-chi earthquake in Central Taiwan. Engineering Geology, 69(1–2), pp.1–13.